Finding on perovskite solar cells may help speed development of green energy technology
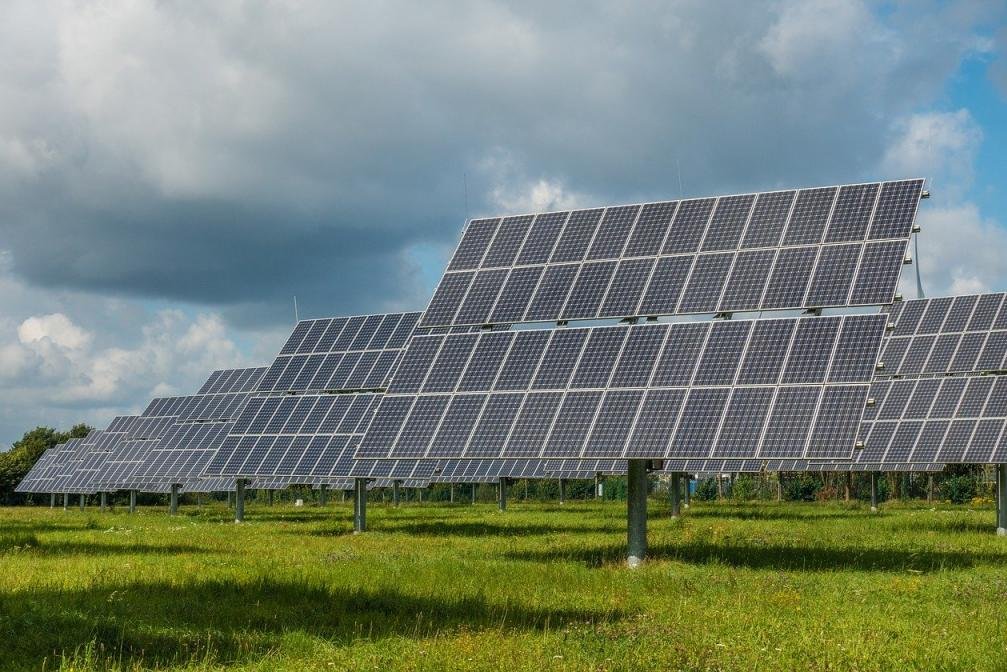
Dec. 6 (UPI) — As researchers look for better materials to harness electricity from the sun, an explanation for the efficiency of one promising material — perovskites — has proven elusive.
Thanks to new research out of Cambridge University, however, scientists no longer are in the dark on perovskite’s virtues.
It turns out that the material’s variegated chemical structure actually guards against the electronic pitfalls associated with similar materials — and could help point the way to improving both it and other materials being developed for green technologies.
The qualities of perovskite, a high-performance solar cell material that turns more of the sun’s rays into electricity, have been demonstrated time and time again — in lab test after lab test.
But despite a decade of laboratory breakthroughs, it wasn’t clear why the material performed as well as it does — until now.
Cambridge University researchers were able to identify perovskite’s nanoscale secrets by surveying its chemical, structural and electronic disorder by using a few different microscopes.
The revelatory observations could accelerate perovskite’s development, allowing scientists to further tweak its composition and integrate the material into commercial solar arrays — and even help light the way for energy research using other materials.
How does it work
“Now, we can basically tell you why it is very good,” lead study author Kyle Frohna, a researcher at Cambridge’s Cavendish Laboratory, told UPI.
To exceed performance standards, solar cell materials typically need to be highly uniform. Crystalline silicon, the material currently used in most commercial solar arrays, is almost perfectly homogenous.
Perovskite’s ability to to absorb a wide spectrum of solar wavelengths and turn them into electricity had previously confounded scientists, primarily because its makeup is the opposite of homogenous.
“The chemical composition of perovskite is more complicated [compared to silicon]. There’s a lot more stuff that goes into them,” Frohna said. “We effectively dissolve all these chemicals into a solvent and then print them out onto a substrate, so you end up with a very complicated landscape.”
This relatively imprecise production process is much more energy efficient, cheaper and easier to scale, which is why scientists expect perovskite to eventually supplant crystalline silicon in the solar cell industry.
Scientists can make a lot of progress through trial and error, without entirely understanding what’s going on beneath the surface. Perovskite is proof of this phenomenon, as are many of the lithium ion battery breakthroughs of the last decade.
But with the impacts of climate change hastening, a pressing need exists for green energy — and the scientific breakthroughs that will enable a carbon-natural energy sector.
As many material scientists can attest, taking a lab breakthrough to market, in which it can have a real-world impact on an industry’s carbon footprint, is extremely difficult. Understanding why and how a breakthrough happened can boost the odds.
“A better understanding makes it more likely that a breakthrough can be fully exploited,” Martin Green, a professor at the University of New South Wales’ Australian Center for Advanced Photovoltaics, who was not involved in the recent study, told UPI in an email.
Freely moving electrons
Solar cell materials work best when their electrons can move freely, without becoming trapped by material defects.
“When there are impurities or defects in the material, they can trap these electrons and cause them to stay there for too long and lose their energy — in the form of heat instead of electricity,” Frohna said.
To learn why perovskite’s incongruities — and resulting electron traps — don’t seem to be all that problematic, researchers used a series of microscopes to more precisely map the material’s structural, chemical and electronic properties.
“First, we used an optical telescope to measure the material’s optical quality, or electronic efficiency,” corresponding author Miguel Anaya, a chemical engineer and research fellow at Cambridge, told UPI.
“For a high quality, efficient material, we expect to see close to one photon emitted for every photon absorbed.”
Then, the researchers used the Diamond Light Source, a synchrotron microscope, to look at the material’s chemical composition and structure.
The results returned by the two microscopes showed that perovskite has two types of disorder working in parallel — chemical disorder and electronic disorder.
Scientists determined perovskite’s chemical disorder creates structural gradients that cause electrons to gravitate toward the parts of the material that have the fewest electronic defects.
Basically, perovskite’s weakness also is a strength.
“Defects are everywhere in these types of samples, but by inducing the electron to find a place in the material that is high-quality, electronically speaking, the chemical disorder masks the effects of the electronic disorder,” Anaya said.
Future research and development
The research team hopes that its illuminating work ultimately will grease perovskite’s path from lab to market.
“Rather than fumbling around in the dark, maybe now we can make more rational decisions about how to modulate these heterogeneous landscapes to suit our purposes even better,” Frohna said.
Frohna and Anaya also said they plan to repeat their work by using fully assembled solar cells, as opposed to a naked perovskite substrate.
Eventually, the team hopes to find ways to identify analytical and computational shortcuts that would allow them to measure the interplay between electronic and chemical disorder without the painstaking process of acquiring and comparing the results from multiple microscopes.
Even farther down the line, the researchers said, it may be possible to use nanoscale observations like theirs for algorithms that could predict how materials will perform inside solar cells or batteries — a breakthrough that could truly streamline the scientific process.
“To get that point we are going to new gather data from a huge amount of materials of with different compositions and qualities, so that you have a catalogue of results to draw from,” Anaya said.